Media
Recovering Lossless Propagation:
HKU Physicists Overcoming Optical Loss in Polariton System
with Synthetic Complex Frequency Waves
24 Jan 2024
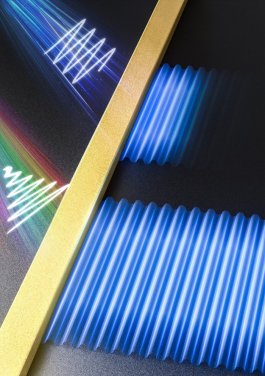
Figure 1. Schematic of polariton propagations under real frequency and synthesized complex frequency excitation. While polariton waves at real frequencies have limited propagation distance, combining propagation waves from different real frequencies based on complex frequencies of incidence can achieve nearly lossless propagation.

Figure 2. 1D Polariton propagation (from left to right) using hBN film operating at optical frequency. (a) Real frequency images show obvious decay field profile at propagation direction. (b) Complex frequency measurements provide almost non-dissipative propagation behavior.
(Figures adapted from Nature Materials, 2024, doi.org/10.1038/s41563-023-01787-8)
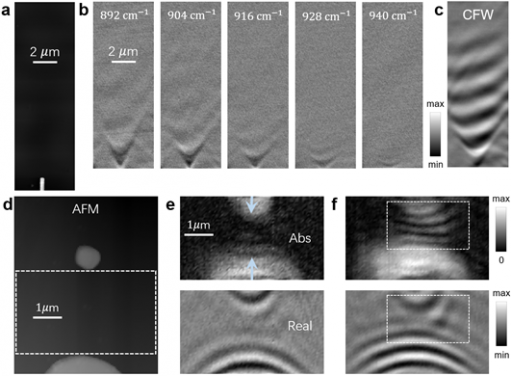
Figure 3. Hyperbolic phonon polariton and elliptical phonon polariton propagation on α-MoO3 film. (a) AFM of an antenna placed on the α-MoO3 film. (b) Real frequency measurements of hyperbolic polariton in different real frequencies. (c) Complex frequency measurement provides an ultra-long distance propagation behavior. (d) AFM of two different spaced gold antennas. (e) The amplitude and real part of the measurements at real frequency f=990cm-1. (f) The amplitude and real part of measurements at complex frequency f=(990-2i)cm-1.
(Figures adapted from Nature Materials, 2024, doi.org/10.1038/s41563-023-01787-8)
A collaborative research team co-led by Professor Shuang ZHANG, the Interim Head of the Department of Physics, The University of Hong Kong (HKU), along with Professor Qing DAI from National Center for Nanoscience and Technology, China, has introduced a solution to a prevalent issue in the realm of nanophotonics – the study of light at an extremely small scale. Their findings, recently published in the prestigious academic journal Nature Materials, propose a synthetic complex frequency wave (CFW) approach to address optical loss in polariton propagation. These findings offer practical solutions such as more efficient light-based devices for faster and more compact data storage and processing in devices such as computer chips and data storage devices, and improved accuracy in sensors, imaging techniques, and security systems.
Surface plasmon polaritons and phonon polaritons offer advantages such as efficient energy storage, local field enhancement, and high sensitivities, benefitting from their ability to confine light at small scales. However, their practical applications are hindered by the issue of ohmic loss, which causes energy dissipation when interacting with natural materials.
Over the past three decades, this limitation has impeded progress in nanophotonics for sensing, superimaging, and nanophotonic circuits. Overcoming ohmic loss would significantly enhance device performance, enabling advancement in sensing technology, high-resolution imaging, and advanced nanophotonic circuits.
Professor Shuang Zhang, corresponding author of the paper, explained the research focus, ‘To address the optical loss challenge in key applications, we have put forward a practical solution. By employing a novel synthetic complex wave excitation, we can achieve virtual gain and counteract the intrinsic loss of the polariton system. To validate this approach, we applied it to the phonon polariton propagation system and observed a significant improvement in polariton propagation.’
‘We demonstrated our approach by conducting experiments using phonon polariton material, such as hBN and MoO3, in the optical frequency range. As expected, we obtained nearly lossless propagation distance consistent with our theoretical predictions,’ added Dr Fuxin GUAN, the paper’s first author and a Postdoctoral Fellow at the Department of Physics at HKU.
Multi-frequency approach to overcome optical loss
In this research, the team developed a novel multiple-frequency approach to address energy loss in polariton propagation. They used a special type of wave called ‘complex frequency waves’ to achieve virtual gain and compensate for the loss in an optical system. While a regular wave maintains a constant amplitude or intensity over time, a complex frequency wave exhibits both oscillation and amplification simultaneously. This characteristic allows for a more comprehensive representation of wave behaviour and enables compensation for energy loss.
While frequency is commonly perceived as a real number, it can also have an imaginary part. This imaginary part tells us how the wave either gets stronger or weaker over time. Waves with a complex frequency featuring a negative (positive) imaginary part decay (amplify) over time. However, directly carrying our measurement under the excitation of complex frequency waves in optics is challenging because it requires complex time-gated measurements. To overcome this, the researchers employed the Fourier Transformation mathematical tool to break down a truncated complex frequency wave (CFW) into multiple components with individual frequencies.
Just like when you are cooking and need a specific ingredient that is hard to find, the researchers used a similar idea. They broke down the complex frequency waves into simpler components, like using substitute ingredients in a recipe. Each component represented a different aspect of the wave. It is like creating a delicious dish by using substitute ingredients to get the desired flavour. By measuring these components at different frequencies and combining the data, they reconstructed the behaviour of the system illuminated by the complex frequency wave. This helped them understand and compensate for the energy loss. This approach greatly simplifies the practical implementation of CFWs in different applications, including polariton propagation and superimaging. By conducting optical measurements at different real frequencies with a fixed interval, it becomes feasible to construct the optical response of the system at a complex frequency. This is achieved by mathematically combining the optical responses obtained at different real frequencies.
Professor Qing DAI, the National Center for Nanoscience and Technology and another corresponding author of the paper, stated that this work has provided a practical solution to address the long-standing issue of optical loss in nanophotonics. He highlighted the significance of the synthesised complex-frequency method, stating that it can be easily applied to various other applications like molecular sensing and nanophotonic integrated circuits. He further emphasised that ‘this method is remarkable and universally applicable, as it can also be utilised to address loss in other wave systems, including sound waves, elastic waves, and quantum waves, thereby enhancing the quality of imaging to unprecedented levels.’
This work was supported by the New Cornerstone Science Foundation, the Research Grants Council of Hong Kong.
The journal paper can be accessed at the following link: ‘Compensating losses in polariton propagation with synthesized complex frequency excitation’, Nature Materials. https://www.nature.com/articles/s41563-023-01787-8
More information about Professor Shuang Zhang: https://shorturl.at/efCN1
For media enquiries, please contact Ms Casey To, External Relations Officer (tel: 3917 4948; email: caseyto@hku.hk / Ms Cindy Chan, Assistant Director of Communications of HKU, Faculty of Science (tel: 39175286; email: cindycst@hku.hk).
Images download and captions: https://www.scifac.hku.hk/press
Supplementary Note: Experimental Demonstration
As a proof of concept, the team started with phonon polaritons (PhPs) propagation at optical frequencies about 1450 cm-1 using hBN films. A long gold antenna placed on the hBN film is used to launch the 1D PhPs. The field distributions of the two real frequencies and the two complex frequencies are displayed in Fig. 2a and 2b, respectively. The experimental results demonstrate that while the propagation at the real frequencies suffers strong attenuation, the polariton at the complex frequencies experiences almost no decay along the propagation.
The team further applied the complex frequency approach to investigate the more complicated field distributions supported by a thin film of van der Waals crystal α-MoO3, which is highly anisotropic and supports natural in-plane hyperbolic polaritons. A metal antenna as an excitation source is placed on the α-MoO3 film as shown in Fig. 3a. The field distribution variation exhibits a characteristic hyperbolic propagation behaviour with a concave wavefront see Fig. 3b. With the increase of the frequency, the wavelength decreases with a stronger field confinement, and meanwhile, the propagation becomes more attenuated. All of these real frequency plots are combined according to the ratio of complex frequency to obtain the complex frequency result in Fig. 3c.
The team finally investigated the interference behavior of PhPs using the complex frequency approach. Two circular antennas with different diameters are fabricated on the MoO3 film to excite the phonon polaritons, as shown in Fig. 3d. While the real frequency plots cannot show clear interference fringes, as shown in Fig. 3e, the complex frequency plots of clear interference fringes can be synthesised after combining the results of different real frequencies and as shown in Fig. 3f.